Research Interests
Development of simulation methods
We develop classical and quantum simulation methods. This includes tools for nuclear quantum effects that are useful for studying enzyme catalysis. This entails development of new path-integral methods for the simulations of zero-point energy and tunneling effects in condensed phase environments. Several new methods are being developed and are incorporated into simulation platforms for enzymatic reactions (e.g. CHARMM – www.charmm.org). The simulations tools are typically used in conjunction with hybrid Quantum Mechanics/Molecular Mechanics (QM/MM) methods.
Enzyme reactions and protein dynamics
We study enzyme assisted catalysis using computer simulation and modeling techniques. We employ hybrid Quantum Mechanics/Molecular Mechanics (QM/MM) methods to obtain accurate chemical, thermodynamic, and kinetic properties of the enzyme. Together with our experimental collaborators we attempt to bridge gaps between theory and experiment to derive a detailed understanding of enzyme function.
In our group we currently focus mainly on the following enzymes that are involved in hydrogen transfer reactions:
DHFR: Dihydrofolate reductase (DHFR) is a small monomer, which catalyzes the reduction of dihydrofolate (DHF) to tetrahydrofolate (THF) in the presence of the cofactor nicotinamide adenine dinucleotide phosphate (NADPH).
FDH: Formate dehydrogenase (FDH) is a dimer, which catalyzes the NAD+-dependent oxidation of formate to carbon dioxide.
Natural product synthesis
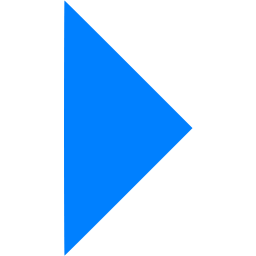


Enzyme Catalysis for Bio-Synthesis of Terpenes
Terpenes as Biofuels: Sustainable and biologically feasible fuels
Protein-ligand docking

We are developing novel hybrid Quantum Mechanics/Molecular Mechanics (QM/MM) docking methods within the CHARMM simulation platform. The great advantage of developing a docking platform within such a general simulation platform is that all the potentials available within CHARMM are naturally also available to the docking code. We particularly focus on developing docking strategies that are suitable for enzymatic systems. Our docking platform allows in depth understanding of molecular recognition in enzymes.
In-silico design of Li-ion batteries
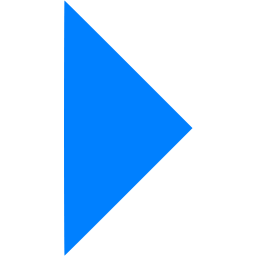
Li-ion rechargeable batteries are promising candidates as portable and durable energy sources, due to their high energy density and power density. In spite of Li-ion batteries (LIB) being the leading battery technology today, there is much room for improvement. Indeed, there are challenges to overcome, such as capacity fading and stability in LIB. In our group, we investigate basic properties, such as electronic, magnetic, mechanical, and electrochemical properties of different cathode materials. Understanding these properties will advance our ability to design novel materials for LIB. The cathode materials being studied include layered oxides, spinel oxides, olivine phosphates, and more. To study these cathodes, we use density functional theory (DFT), as well as a range of classical simulation techniques.
Design of Mg-ion Batteries

Recent rapid technological improvements has led to a quest for alternative energy sources. Energy storage systems such as batteries occupy a central role in driving the electrification of our societies. One family of batteries that has attracted considerable attention in recent decades is Magnesium ion batteries, as they are considered a potential replacement for lithium in rechargeable batteries.
Fuel Cells
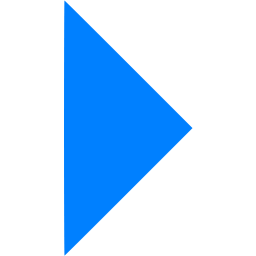
The projected limited supply of energy is one of the biggest problems of this century. In recent years, with an increase in global population density, we see a surge in pollution and a concomitant damage to the natural environment. Without a doubt, an alternative way to produce energy must be developed. One of the most promising renewable energy technologies today is fuel cells. Fuel cells are devices that convert chemical energy to electrical energy by redox reactions that occur at the anode and cathode. In our group we study bio-inspired fuel cell materials using atomistic density functional theory (DFT) calculations.
Collaborations
Terpenes represent the largest class of natural products currently known. These natural compounds are derived from a few linear precursors, such as geranyl diphosphate (GPP, C10), farnesyl diphosphate (FPP, C15) and geranylgeranyl diphosphate (GGPP, C20). Terpenoids, which make up 60% of all natural products, have diverse biological functions that are widespread across all three domains of life.
Prof. Amnon Kohen (University of Iowa) - https://chem.uiowa.edu/kohen-research-group
Prof. Jeroen Dickschat (University of Bonn) - https://www.chemie.uni-bonn.de/Jeroen
Prof. Doron Aurbach (BIU) - http://ch.biu.ac.il/aurbach
Prof. Lior Elbaz (BIU) - http://ch.biu.ac.il/node/821
Prof. Bilha Fischer (BIU) - http://bilhafischerlab.wixsite.com/bilha-fischer/contact
Prof. David Zitoun (BIU) - http://www.zitounlab.com
Prof. Arie Zaban (BIU) - www.zabanlab.com
Dr. Yoni Toker (BIU) - http://yonitoker.wixsite.com/actionspectroscopy